
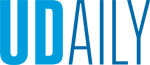

Advancing lung research
Photos by Kathy Atkinson and Evan Krape | Photo illustration by Jeffrey C. Chase February 19, 2025
3D-printed lung model helps researchers study aerosol deposition in the lungs
Respiratory diseases are a challenging problem to treat. Inhalable medicines are a promising solution that depend on the ability to deliver tiny particles known as aerosols to the correct location in the lungs at the correct dosage.
How effectively this works can get complicated, depending on the drug, delivery method and patient. This is because it is difficult to predict just how much medicine gets in and where it goes in the lung. Similar challenges exist when thinking about measuring an inhaled environmental exposure, say to particles of asbestos or a toxin like smoke.
“If it's something environmental and toxic that we're worried about, knowing how far and how deep in the lung it goes is important,” said Catherine Fromen, University of Delaware Centennial Associate Professor for Excellence in Research and Education in the Department of Chemical and Biomolecular Engineering. “If it's designing a better pharmaceutical drug for asthma or a respiratory disease, knowing exactly where the inhaled aerosol lands and how deep the medicine can penetrate will predict how well that works.”
Fromen and two UD alumni have developed an adaptable 3D lung model that can replicate realistic breathing maneuvers and offer personalized evaluation of aerosol therapeutics under various breathing conditions. The researchers have submitted a patent application on the invention through UD’s Office of Economic Innovation and Partnerships (OEIP), the unit responsible for managing intellectual property at UD.
In a paper published in the journal Device, Fromen and her team demonstrate how their new 3D lung model can advance understanding of how inhalable medications behave in the upper airways and deeper areas of the lung. This can provide a broader picture on how to predict the effectiveness of inhalable medications in models and computer simulations for different people or age groups. The researchers detail in the paper how they built the 3D structure and what they’ve learned so far.
Valuable research tool
The purpose of the lung is gas exchange. In practice, the lung is often approximated as the size of a tennis court that is exchanging oxygen and carbon dioxide with the bloodstream in our bodies. This is a huge surface area, and that function is critical — if your lungs go down, you're in trouble.
Fromen described this branching lung architecture like a tree that starts with a trunk and branches out into smaller and smaller limbs, ranging in size from a few centimeters in the trachea to about 100 microns (roughly the combined width of two hairs on your head) in the lung’s farthest regions. These branches create a complex network that filters aerosols as they travel through the lung. Just as tree branches end in leaves, the lung’s branches culminate in delicate, leaf-like structures called alveoli, where gases are exchanged.
“Those alveoli in the deeper airways make the surface area that provides this necessary gas exchange, so you don't want environmental things getting in there where they can damage these sensitive, finer structures,” said Fromen, who has a joint appointment in biomedical engineering.
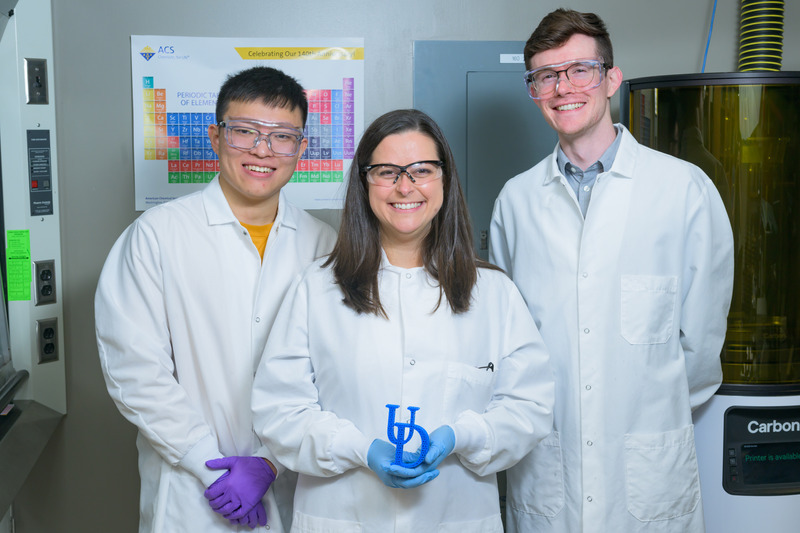
Mimicking the complex structure and function of the lung in a lab setting is inherently challenging. The UD-developed 3D lung model is unique in several ways. First, the model breathes in the same cyclic motion as an actual lung. That’s key, Fromen said. The model also contains lattice structures to represent the entire volume and surface area of a lung. These lattices, made possible through 3D printing, are a critical innovation, enabling precise design to mimic the lung's filtering processes without needing to recreate its full biological complexity.
“There's nothing currently out there that has both of these features,” she explained. “This means that we can look at the entire dosage of an inhaled medicine. We can look at exposure over time, and we can capture what happens when you inhale the medication and where the medicine deposits, as well as what gets exhaled as you breathe.”
The testing process
Testing how far an aerosol or environmental particle travels inside the 3D lung model is a multi-step process. The exposure of the model to the aerosol only takes about five minutes, but the analysis is time-consuming. The researchers add fluorescent molecules to the solution being tested to track where the particles deposit inside the model’s 150 different parts.
“We wash each part and rinse away everything that deposits. The fluorescence is just a molecule in the solution. When it deposits, we know the concentration of that, so, when we rinse it out, we can measure how much fluorescence was recovered,” Fromen said.
This data allows them to create a heat map of where the aerosols deposit throughout the lung model’s airways, which then can be validated against benchmarked clinical data for where such aerosols would be expected to go in a human under similar conditions.
The team’s current model matches a healthy person under sitting/breathing conditions for a single aerosol size, but Fromen’s team is working to ensure the model is versatile across a much broader range of conditions.
“An asthma attack, exercise, cystic fibrosis, chronic obstructive pulmonary disorder (COPD) — all those things are going to really affect where aerosols deposit. We want to make sure our model can capture those differences,” Fromen said.
The ability to look at specific disease features, say, narrowing of the airways or accumulation of mucus, could one day contribute to more personalized care. For example, perhaps a patient might need longer doses of medicine because the medication is not getting saturated in the body site, or possibly they need a redesigned patient inhaler, so it targets a specific region. This is currently difficult to implement, but the UD-developed model provides a baseline tool for asking those questions.
“This is important because, right now, inhaled pharmaceutics are designed with a one-size-fits-all approach. But someone who has severe COPD, for example, is going to breathe very differently and have a very different lung structure than someone who is healthy,” Fromen said.
Additionally, many inhaled medicines fail clinical trials for unknown reasons. When it doesn't work, researchers wonder, is it the molecule that isn’t effective, was the formulation flawed? Or did the molecule fail to accumulate at a certain level at its target in the lungs?
According to Fromen, clinical trials typically focus on whether a medication results in measurable improvement in a disease, while the UD-developed tool can provide deeper insights. It can determine whether the aerosol got where it needed to go in the first place, and in the right amount, potentially saving time and effort in formulation development and reducing setbacks during clinical trials.
The researchers shared their design and methods in an open-source format, in hopes others will adopt the UD-developed technique.
“Making it accessible to other researchers opens the door for impactful collaborations,” Fromen said. “Clinicians can provide priority patient profiles for us to model, while pharmaceutical developers could integrate the model into their workflows to optimize treatments for specific respiratory conditions.”
Contact Us
Have a UDaily story idea?
Contact us at ocm@udel.edu
Members of the press
Contact us at 302-831-NEWS or visit the Media Relations website