
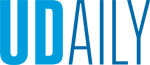
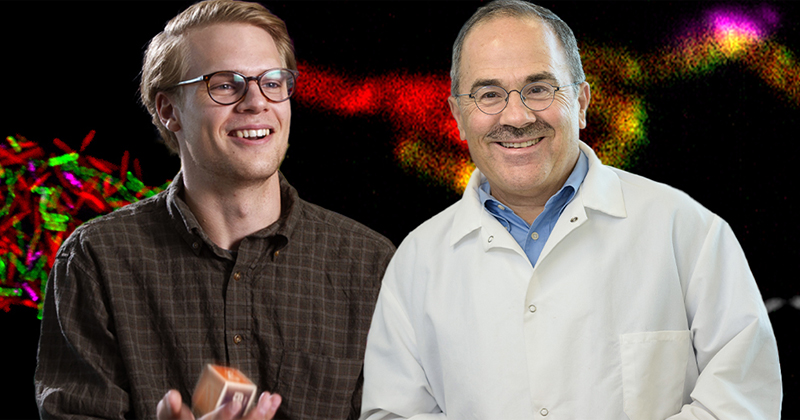
Observing microbial communities
Photos courtesy of Terry Papoutsakis and John Hill September 11, 2024
UD researchers develop new technique to watch microbial populations interact
Microscopic organisms such as bacteria and fungi live together in neighborhoods known as microbial consortia. Some of these neighborhoods naturally exist in soils, food, water, and even the human gut and skin. Others are engineered together in order to degrade pollutants or to produce or convert chemicals.
Scientists examine microbial consortia to understand and dissect the complex interactions that occur between the tiny organisms in order to explain their observable behavior and design new, beneficial consortia. Researchers have always assumed that microbial interactions take place through release of select chemicals into their environmental milieu or the culture medium. Other cells could uptake those chemicals, but the exchange was always thought to occur without exchanging cellular material between the different cells in the consortium.
“But there is growing evidence that different cells may exchange material en masse,” said Eleftherios “Terry” Papoutsakis, Unidel Eugene du Pont Chair Professor in the College of Engineering’s Department of Chemical and Biomolecular Engineering. “We need tools to examine such possibilities.”
This type of unexpected exchange of cellular material in groups, or “en masse,” alters the behavior of the individual members of the consortium and may result in the development of new synthetic capabilities and metabolic activities as well as in energy savings. Such alterations can be used for many practical applications to benefit sustainability and the environment.
University of Delaware researchers have developed a new technique to observe how cell populations interact in these synthetic (human-made) microbial communities. The researchers published their findings in the journal, mSystems. The findings highlight a new method to get clues into microorganisms’ behavior and their population dynamics. The bacterial cultures examined may also serve as a tool to help fight climate change.
A new diagnostic tool
A few years ago, UD researchers were growing two species of bacteria together when they noticed the cells doing something unusual.
“The cells would come together at their poles and then exchange a lot of material originating from inside of the cytoplasm,” said John Hill, a fourth-year doctoral candidate in the UD Department of Chemical and Biomolecular Engineering.
Cytoplasm is a thick liquid inside cells that contains all the genetic information and cellular machinery that allows the cells to live and replicate. Hill said the bacteria cells coming together and exchanging this material was unusual because the cells eventually gave rise to hybrid cells — cells containing an amalgam of both organisms’ cytoplasmic material, which hasn’t been seen before.
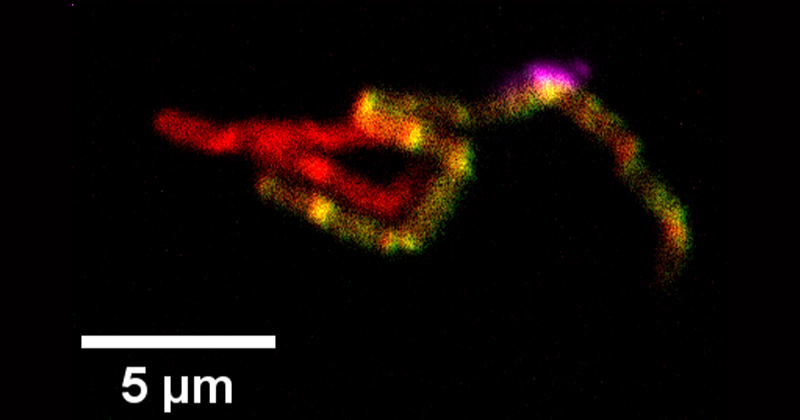
Other researchers had previously shown proteins were being exchanged from one cell to another. But UD researchers had more questions and wanted to take this a step further. They wondered: could microbes’ large cellular machinery be exchanged between cells?
“That would tell us that the bacteria had methods of quickly gaining new cellular functions from their neighbors,” Hill said.
To figure this out, the researchers attached fluorescent tags to the microbes’ ribosomal ribonucleic acid (rRNA), a single-stranded molecule that forms a scaffold for the cell’s ribosome. The ribosome is a large complex that synthesizes the proteins in cells.
A microscope can be used to determine where the fluorescent tag is since it glows “something like a tiny lightbulb,” as Hill likes to describe it. “The cell is completely dark, except this little point of light where the rRNA is,” he said.
The technique the researchers used, called rRNA fluorescence in-situ hybridization (rRNA-FISH), can allow researchers to visualize and track microbes using fluorescent microscopy and flow cytometry. Flow cytometry enables the quantitative examination of a large population of cells in a fast, high-throughput way. While rRNA-FISH has been used for years for species identification, the use of the method here is novel, leads to unexpected findings and facilitates new applications.
The researchers looked at three bacterial cultures of species in particular, Clostridium acetobutylicum, Clostridium ljungdahlii and Clostridium kluyverii, to see how the bacteria were interacting with each other. Each was given their own unique color or fluorescent tag.
The research team adapted some tools to label the ribosomal material, using fluorescent probes with different colors to label the very abundant rRNA of each cell type. They took microscopic images and used flow cytometry to track the colored, fluorescent signals in the cell population and how the signals of the labeled cells changed with time.
“We found some cells that had two different colors,” Hill said, “indicating that those cells had ribosomal material which originated from two different species. The corollary of that is the cells had at some point in time actually exchanged the ribosomal material.”
This means that they contain ribosomes from more than one organism and that therefore, since ribosomal RNA and ribosomes are very large molecules and molecular structures, it shows that each microbe type exchanges cellular material with a different microbe. And does so en masse.
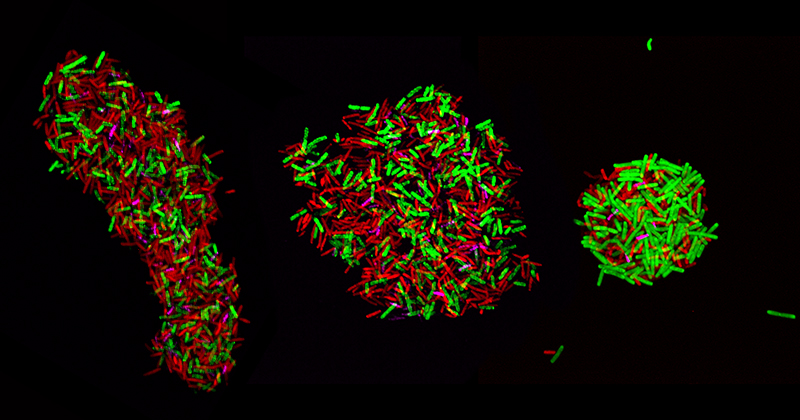
Climate applications
The three bacteria the scientists examined all have a hand in combating climate change — removing carbon dioxide from the atmosphere and reducing the amount of new carbon dioxide entering the atmosphere, which contributes to increasing extreme weather, such as extreme heat, drought, storms and fires.
“Everybody wants to remove CO2 from the atmosphere and convert waste CO2 into useful chemicals,” Papoutsakis said. “It’s like a circular economy. You take your waste and convert it to useful products.”
Each species has their own special role in the consortium. Clostridium acetobutylicum can ferment carbohydrates into useful chemicals that can be used for a broad set of applications, including biofuels. Clostridium ljungdahlii can convert CO2 into organic molecules. Clostridium kluyveri can convert short alcohols and organic acids into longer-chain fatty acids that have many applications in products used in the personal care, health and materials-synthesis sectors.
Hill explained that while Clostridium acetobutylicum’s fermentation process releases CO2, Clostridium ljungdahlii juxtaposes it by eating the CO2 as it is produced. Clostridium kluyveri can, in turn, upgrade all these products into something even more valuable.
“That means we're sort of approaching carbon neutral and even in some cases, carbon negative fermentation, which just means that we're actually able to feed additional CO2 into the system,” Hill said. “But it also means that we're increasing our ability to produce a usable product from the same amount of sugar substrate. It’s better for the process economics, but it also releases less CO2.”
Papoutsakis said he’d like to continue to use rRNA-FISH technology to further understand how cells share cellular material with each other.
“But broadly speaking, we’re going to be using rRNA-FISH as a diagnostic and a synthetic tool to optimize microbial culture conditions,” Papoutsakis said, “and to be able to produce the chemicals we want to produce by fixing CO2. That’s really where we are.”
About the funding
The research, Species-specific ribosomal RNA-FISH identifies interspecies cellular-material exchange, active-cell population dynamics and cellular localization of translation machinery in clostridial cultures and co-cultures, was largely funded by the UD Department of Energy | Advanced Research Projects Agency - Energy (ARPA-E) under contract AR0001505.
Contact Us
Have a UDaily story idea?
Contact us at ocm@udel.edu
Members of the press
Contact us at 302-831-NEWS or visit the Media Relations website